The Zocchi Group: Molecular BioPhysics and Nanoscience
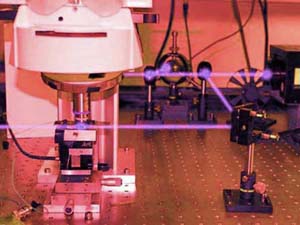
Living matter, at the molecular scale, is different from usual matter. Biological macromolecules deform without breaking, couple reactions to motion, perform tasks. Stretching a point, we may say that at the molecular scale, life is the coupling of chemical reactions to conformational motion (which is to say: life is made of enzymes). We are interested in the essence of life (Indiana Jones’ fashion), therefore in mechano-chemical coupling. We focus on two approaches.
The first is to make new molecules with an embedded function. Constructing artificial mechano – chemical devices is in itself an instrument of inquiry into mechano – chemistry.
The second approach is to develop new nanomechanical and optical techniques with which we study, provoke, perturb, conformational changes of biological macromolecules (enzymes, DNA).
Our experimental approach is materials science and condensed-matter-physics oriented. Coming from the Physics of Complex Systems, we seek properties which display a degree of universality. Where it exists, universality usually translates into a simple mathematical description.
In the end, the mission of the lab is to do beautiful experiments. With luck, discovery may follow.
One area where universal behavior emerges is the mechanical (“rheological”) properties of the folded protein. Our group developed a nanotechnology method (“nanorheology”) which probes deformations of the folded state with sub-Angstrom resolution. Through these measurements we discovered that enzymes are viscoelastic. This is a fundamental materials property of the protein molecule, and is allowing new insight into the mechano-chemical cycle of enzymes.
Short DNA molecules are mechanically similar to semi-flexible rods – at the nanoscale. Our group invented a thermodynamic method to directly measure the elastic energy of a short DNA molecule under stress. We characterized quantitatively a bifurcation in the mechanics of the molecule analogous to the buckling of a macroscopic stick (the Euler instability). These experiments led to a simple analytical expression for DNA bending elasticity in both the linear and nonlinear regimes. They lay the conceptual basis for a more general study of nonlinearity with macromolecules pursued by the group.
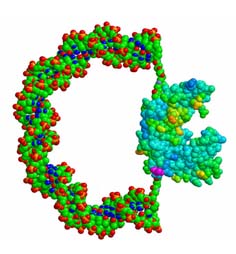
We are interested in controlling enzymes mechanically, another area where universal behavior may emerge. One mechanical component which, unlike nuts and bolts, can be shrunk to the nanoscale is the humble spring. By inserting a “molecular spring” on an enzyme we control the molecule’s conformation, and thus modulate the enzymatic activity. We make new molecules which are both a tool to study mechano-chemical coupling in enzymes and also lay the foundation for a new generation of molecular devices.
DNA is the molecule which encodes the master plan for the cell. Decoding this information, i.e. the process of expressing and controlling genes, involves a variety of conformational changes of DNA caused by protein-DNA interactions. Enzymes are the molecular machines which perform the tasks in the living cell. This includes catalysis, molecular recognition, mechanical motion, and in general energy conversion. Virtually all these tasks involve conformational changes of the protein. Enzyme deformability thus emerges as a fundamental property of living matter at the molecular scale.